Research is ongoing in the following areas of atmospheric chemistry:
- Air/sea fluxes
- Reactive halogens
- Trace gases in polar ice
- Air trapped in polar firn
Air/sea fluxes
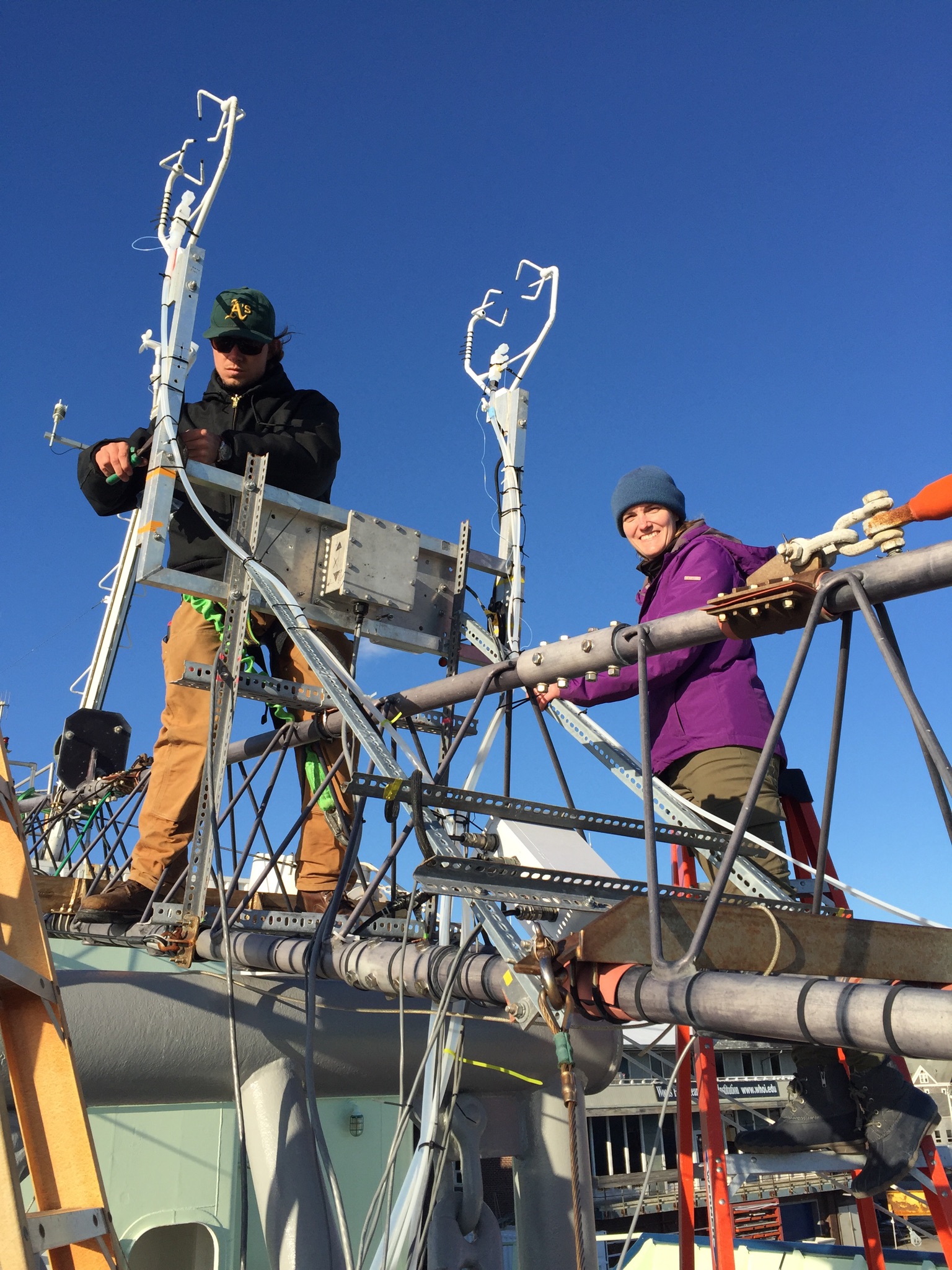
Jack Porter and Mackenzie Grieman prepping the equipment during the mobilization period for the NASA NAAMES cruise. Eddy covariance instruments aid in trace gas air/sea flux measurements.
Air/sea fluxes are a significant component of the global biogeochemical cycles of many climate-active gases. These include greenhouse gases (CO2, CH4, N2O), stratospheric ozone-destroying halocarbons (CH3Br, CH3Cl, CHBr3, etc.), aerosol-forming gases (DMS, NH4) and volatile organic compounds which impact tropospheric photochemistry. The need for a better understanding of the biogeochemical coupling between the surface ocean and atmosphere is widely recognized. Developing predictive models for future climate change requires both knowledge of current ocean/atmosphere fluxes, and more importantly, an understanding of the processes forcing gas exchange, and how they relate to the physical and biological state of the oceans.
This project involves the use of eddy correlation to directly measure trace gas fluxes across the air/sea interface. Eddy correlation requires fast response chemical measurements and simultaneous 3-dimensional winds. We use chemical ionization mass spectrometry to measure dimethylsulfide and acetone fluxes, and infrared gas analyzers to measure CO2 fluxes. This is a collaborative effort involving our laboratory, Scott Miller (SUNY Albany) and Warren De Bruyn (Chapman Univ.).
Our research team has carried out several major cruises including:
- A 2004 study in the equatorial and North Pacific
- A 2006 study in the eastern tropical and South Pacific
- Two cruises during 2007 and 2011 in the North Atlantic
- A 2012 cruise to the Southern Ocean aboard the New Zealand (NIWA) research vessel Tangaroa
- A series of cruises to the North Atlantic between 2015 – 2017 as part of the NASA North Atlantic Aerosols and Ecosystems Study (NAAMES)
We have also carried out coastal pier deployments at Scripps Pier, California and Duck, North Carolina.
Reactive halogens
Transformations involving reactive halogens (chlorine, bromine, and iodine) can dramatically alter atmospheric photochemistry. Stratospheric ozone depletion is the most notable example. In the troposphere, the chemical processing of reactive halogens significantly influences photochemistry over Arctic and Antarctic sea ice, marine kelp beds, salt flats, and coastal cities. In these environments, reactive halogens influence transformations in both vapor and particulate phases, affecting ozone, sulfur, and mercury cycling and the formation of new particles. In polluted air, chorine chemistry leads to the formation of ozone and halogenated organics, both of which are of concern with regard to human health.
There are several indirect lines of evidence suggesting that halogen chemistry can be important over the oceans. If so, this could affect the lifetime and reaction pathways of many climate-active gases, like CH4 and DMS. However, there are few direct measurements of these reactive chemicals in marine air, and we have a limited understanding of how they are produced in the atmosphere. Our laboratory is developing measurement techniques to detect reactive chlorine, bromine, and iodine gases and their precursors, and carrying out field studies in various marine environments. The analytical techniques we use are based on chemical ionization mass spectrometry.
An example is the 2007 field study at Cape Verde Atmospheric Observatory, an island research station off the coast of Africa in the tropical eastern Atlantic ocean. Measurements of Cl2 at Cape Verde demonstrated that reactive chlorine is produced in marine air as polluted air masses pass over the ocean. This appears to be caused by acidification of marine aerosols by pollutant nitric and sulfuric acids. This was a surprising discovery, and it was the first time this phenomenon had been directly observed. We recently returned to Cape Verde in 2009 to test new analytical techniques for measuring both Cl2 and HOCl. This study included the first -ever measurements of tropospheric HOCl. The study showed unexpectedly high levels of HOCl that are not explained by current photochemical models.
Trace gases in polar ice cores
The gases trapped in polar ice cores have proven to be a unique and invaluable archive for investigating paleoatmospheric variability of gases such as carbon dioxide, oxygen, methane, and nitrous oxide. The ice archive has barely been tapped for trace gases of lower abundance, many of which have a major impact on climate and atmospheric chemistry. The diverse array of low-level trace gases in ice bubbles potentially contains a wealth of information about biogeochemical cycles that control trace gas emissions and loss.
In this study, we focus primarily on the naturally-occurring halocarbons methyl chloride (CH3Cl) and methyl bromide (CH3Br), a sulfur gas (OCS), and on hydrocarbons (particularly ethane) which all play a role in global atmospheric chemistry and climate. Ice core records of records of paleoatmospheric levels of these gases will help to show the anthropogenic impact on the atmospheric loading of these compounds, reveal the range of their natural variability in the atmosphere on a variety of time scales, and provide insight into the relationship between atmospheric trace gas levels and climatic change.
The measurements involve shredding or melting the ice core samples at low temperature under vacuum, releasing the air trapped in ancient bubbles. The air is collected, and analyzed by gas chromatography with mass spectrometric detection. Because the amount of gas evolved from ice core samples is small, the analysis requires high sensitivity and very low levels of contamination. To date, we have made measurements on Antarctic ice cores from South Pole, Siple Dome, WAIS Divide, Taylor Dome, and Vostok and on ice cores from Summit, Greenland. We are currently making measurements on deep Antarctic ice cores to examine the trace gas composition of the glacial atmosphere. Our next venture is analysis of the newly drilled South Pole Ice Core which was drilled between 2014-2017. This project was funded by the National Science Foundation and is a joint collaboration between the University of California, Irvine, University of Washington, and the University of New Hampshire.

The South Pole Ice Core camp and drilling tent during the first field season (2014-2015). Photo by Mindy Nicewonger
Air trapped in polar firn
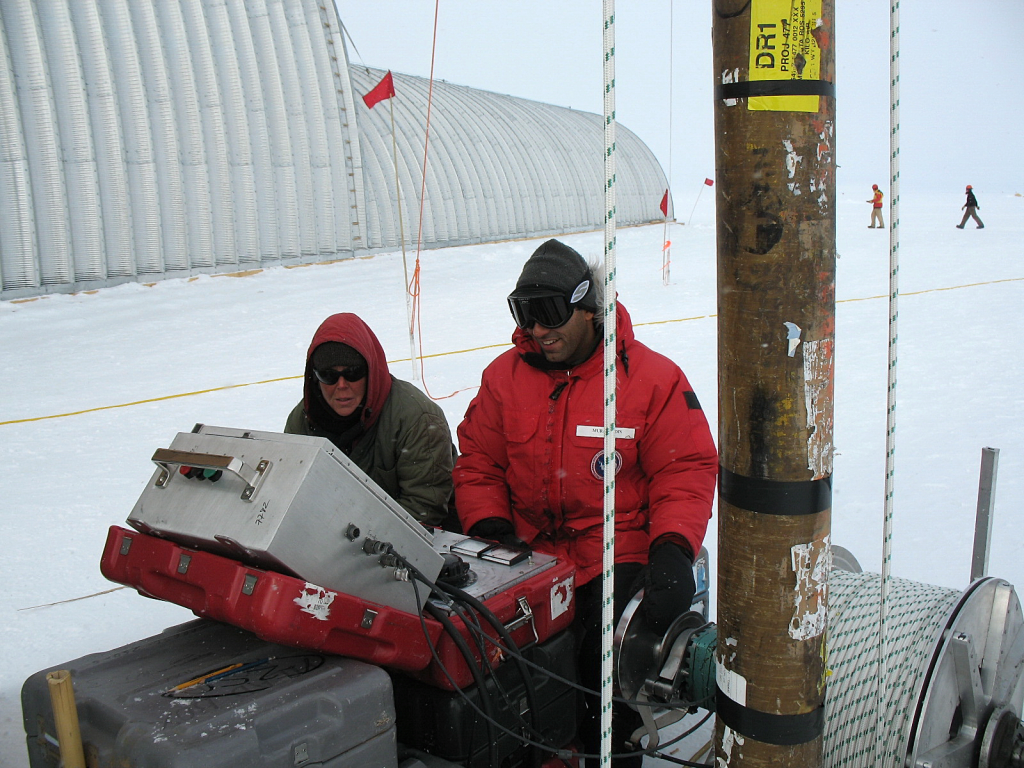
Murat Aydin at WAIS Divide in 2008 collecting firn air samples.
Snow deposited on the Greenland and Antarctic ice sheets gradually metamorphoses into ice as it is buried beneath new layers of fresh snow. During that process, air can diffuse through the pores between ice crystals. This “firn air” increases in age with increasing depth, and it can be used as an archive for the atmosphere. Depending on the location, firn air ages range from a few years to about 100 years. At the base of the firn layer, at the firn/ice transition, the air is trapped and eventually enclosed in bubbles. We are studying the trace gas composition of firn air in order to learn about changes in atmospheric chemistry over the past century, and to better understand the process by which air is trapped in ice core bubbles.
Over the past few years, we have analyzed firn air from several sites in Greenland and Antarctica. In 2008, Murat Aydin lead a team to South Pole, with the goal of obtaining a record of atmospheric ethane over the past century. These firn air studies are collaborative efforts with colleagues from Penn State Univ., Bowdoin College, Scripps Institute of Oceanography, and NOAA. The ethane record shows a dramatic increase over the first half of the 20th century, followed by a surprising decline to modern levels. These data indicate that fossil fuel hydrocarbon emissions declined even as production and use increased. This decline was most likely due to improvements in efficiency of industrial practices. The timing of the decline is strikingly similar to the late 20th century decline in methane growth rates, suggesting that decreasing fossil fuel emissions is the common cause. Read the full publication here.